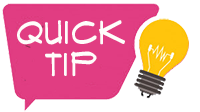
Objectives
Students will know how plants are able to remove nitrate pollution, and will be able to compare differences in nitrate uptake by aquatic or terrestrial plants.
Overview
Rating:
(1) Students will review background information on how excess nitrates can create low dissolved oxygen levels and how aquatic plants can remove or filter excess nitrates.
(2) Students will set up plant/fertilizer ecosystems in bottles or pots and measure nitrates for at least a week.
(3) Based on this information, students will make inferences and conclusions about the benefits of wetland plants.
Materials
- Computer access with Google Earth or online access to view a global map of oceanic dead zones: http://bit.ly/1ggJpik
- Computer access and speakers to show a short CBS news video clip on dead zones: http://cbsn.ws/1a0G5Xv .
Aquatic Ecosystem | Terrestrial Ecosystem |
Mason jars or other containers (5-10 per group) | Plant pots, soil, radish, lettuce, or bean seeds (3 per pot, remove two weakest after germination) |
Pond or treated aquarium water | Water |
Measuring spoons | Graduated cylinder |
Aquatic Plants: rooted (add sand/gravel mix) or floating | Grow lights-optional |
Fertilizer | |
Buckets: to prepare solutions of fertilizer, making it easier to add the treatment throughout the experimental process | |
Nitrate/nitrite test kits – WaterWorks Nitrate/Nitrite Test Strips are inexpensive and work well (sold by Nasco); or use a colorimeter or titration kit |
Procedure
Preparation:
- 1 half gallon of fertilizer and distilled water mix that has been sitting indoors in sun for 2 weeks (double the dose suggested by Miracle Gro liquid fertilizer, the initial reading should be around 50 ppm)
- Order plants or seeds.
- Assemble materials
Engage
Ask students what comes to mind when they hear the term “dead zone.”
A dead zone is an area of the ocean where there is too little oxygen to support marine life. These zones are created when excessive nutrients are added to the water, accelerating the growth of both algae (oxygen producers) and bacteria (oxygen consumers). However, the algae require light to grow, so as the microbes’ abundance increases, they shade each other out and decrease available light to organisms below. This means that the algae on top get light and can continue to grow (and thus produce oxygen at the surface), but beneath, the shaded-out algae die, further feeding oxygen-consuming bacteria and creating large areas where the oxygen plummets.
A short CBS news video explains the problem in the Gulf of Mexico: http://cbsn.ws/1a0G5Xv. According to recent work by Diaz & Rosenberg, the number of dead zones has increased by 33% between 1995 and 2007. There are currently more than 405 dead zones, affecting an area of 95,000 square miles (about the size of New Zealand). To show students the extent of the problem, open Google Earth and open the “Layers” pane. Navigate to the Ocean/State of the Ocean/Dead Zones. Each dead-zone location in Google Earth is represented by a skeletal fish icon and includes data on the nature of the dead zone, its size, date, impact on fisheries, and impact on deep-water ecosystems. Make sure to zoom in along the coasts to see just how many skeletal fish there are – at the global view the icons are too small to see. Alternatively, visit http://bit.ly/1ggJpik to see a Google Map image of dead zones hosted by the Virginia Institute of Marine Science.
Explore:
Hand out the worksheet. Ask students to answer the questions in Part 1 of the worksheet (a graph of nitrate-nitrogen changes in a Hudson River tidal marsh). First, ask students to explain the trend in nitrate-nitrogen. Students should notice that the nitrate levels go down over time, as the water leaves the marsh. Ask students: What do you need to know in order to decide what caused the change in nitrate-nitrogen in the marsh? Students should ask questions such as:
- Does this pattern hold true for all ebbing tides?
- What lives in the marsh?
- What type of vegetation is in the marsh?
- What time of year did this take place?
- Depending on the type of vegetation, does nitrate removal change?
- Were there any nitrogen inputs nearby, like a sewage treatment plant?
- Are there other organisms that could be using the nitrogen?
- How much nitrogen can plants remove?
Explain to students that they will be conducting their own investigation to learn about nitrogen changes in a tidal marsh and will return to these data at the end of the investigation. Do not answer the question for the students at this point. Students may have no idea how to answer the question, but by the end of the experiment, they should realize that plants can remove nitrogen from the water. In the wetlands along the Hudson River, the tides bring water with high nitrate-nitrogen into the marshes, and over the course of the six hour tide, the plants remove much of the nutrient, returning water that has lower nutrient levels to the main stem of the river.
Students will set up and carry out the experiment in the worksheet to test the role of plants in removing nitrates. There are several variations of this experiment. Options include:
1) Investigating the role of different wetland plants. Students will need several types of wetland plants, jars or 2-liter soda bottles, and a soil mixture. These can be purchased from Crystal Creek Pond Supply; cattails, arrow arum, and arrowhead cost around $5 per plant at the time of this writing. Remind students to set up controls. Results are optimum at 2-3 weeks.
2) Investigating the role of aquatic plants. Students will need different types of aquatic plants that are commonly available in ponds and/or pet supply stores (elodea, duckweed). Be careful about purchasing plants that may be invasive. To properly dispose of water with non-native plants: 1) Boil the water for at least 60 seconds. 2) Filter it. Pour water down the drain. Compost or trash plant debris. Remind students to set up controls. Results are optimum within one week.
3) Investigating the role of terrestrial plants on soil nitrogen. Students will need several types of seeds (lettuce, radish, and beans germinate quickly), soil, and containers. Remind students to set up controls. Results are optimum at 2-3 weeks.
Ideally, students will work in small groups and test several different plants; alternatively, you can reduce the amount of materials needed by having a control group, a second group that tests just one type of plant, etc. While students may just measure the change in nitrate-nitrogen in the water, they might also measure plant growth, change in mass, other visual changes, dissolved oxygen levels, etc.
This is designed as an inquiry investigation in which students design their own procedure, but some students may need more scaffolding. Here is a sample procedure for the aquatic plants investigation. This procedure is designed to address the question: Do different aquatic plants affect nitrate levels differently?
1. Cut off the top portion of nine 2-liter plastic bottles if you are using two types of plants.
2. Pour 8 oz. of sand into the bottom of each of the bottles, and then pour 4 oz. of rock substrate on top of the layer of sand. Pour enough water into each bottle to cover the substrate by 2 inches. Mark the water line with a short black line on the outside of the bottle. This will make it easier when you add water to the bottles later.
3. Label the bottles- three of treatment 1 with plant type 1 (e.g. P1-1,P1-2,P1-3), three of treatment 2 with plant type 2 (e.g. P2-1,P2-2,P2-3), and three controls with no plants (e.g. C1, C2, C3).
4. In each of three replicate treatment bottles (P11-3), place four individuals of one plant type (recommended: Dwarf sagittaria, Elodea, or plants collected from local ponds/wetlands). Gently push the roots down into the rock layer to stabilize the plant. Do the same for the other plant type (P21-3), creating a total of three replicates of each plant.
*Note: Many commonly sold aquarium plants are invasive, such as Cabomba caroliniana, or fanwort. Use caution when using these plants, and dispose of them by first boiling them in their water, then throwing them in the trash when finished with your experiment!
5. Prepare a high liquid fertilizer concentration by doubling the recommended dose on the fertilizer container.
6. Add the desired amount of fertilizer to each bottle.
8. Place the bottles together in an area which receives the same amount of light and temperature. *Be sure to randomize their order. Certain locations often receive more light than others, and you don’t want this to be a systematic bias (i.e. All the P1’s receive more light, because they are closest to the window.)
9. Test the nitrate-nitrogen levels of the water in each container for 1-3 weeks. Record all data & observations in your data table.
Sample data are provided in the answer key.
In an “ideal experiment” which mimics the actual freshwater tidal wetland, you would expect to see graminoid plants like cattails removing more nitrate-nitrogen than the broadleaf plants (arrowhead and arrow arum, in this case). However, whatever your results, this is a good chance to discuss experimental design, induced (human) error, and real, natural variability within the ecosystem.
Explain
For background on nitrogen and the nitrogen cycle, see the “Nitrogen in Ecosystems” lesson plan, which includes an ‘overview nitrogen’ game.
Nitrogen exists in many different forms. It is essential for organisms, because it is part of genetic material (DNA, RNA, amino acids), proteins, and important molecules like chlorophyll. All organisms require nitrogen in order to live. Most nitrogen (N) exists as dinitrogen (N2) gas in the atmosphere, but plants can’t use it in this form. It has to be “fixed,” or converted to an inorganic compound such as ammonium (NH4+) and nitrate (NO3-). This can be done naturally by lightning (transforming it into nitrate which then rains onto soil) or bacteria, or by humans through the manufacture of fertilizer and the combustion of fossil fuels. Some nitrogen-fixing bacteria live in the soil; others live in the root nodules of certain kinds of plants. Rhizobia are a group of bacteria that live symbiotically with legumes, providing nitrogen while receiving sugars. When plants primarily take up nitrate (NO3-), they use it to make proteins, amino acids, and nucleic acids after reducing it to nitrite (NO2-) and then ammonium (NH4+). Animals receive the nitrogen they need for metabolism, reproduction, and growth by consuming living or dead organic matter, which already contains nitrogen in a variety of forms.
In more biogeochemical words, when an animal or plant dies, or an animal excretes, the nitrogen is ‘mineralized’ or changed from an organic (i.e. proteins and DNA) form to an inorganic form, such as ammonia (NH3) through physical and biological (decomposing) processes. Ammonia (NH3) is then oxidized into ammonium (NH4+), then nitrite (NO2-), and nitrate (NO3-) through the process of nitrification. The last step in the ‘cycle’ is denitrification, when nitrites are reduced back into nitrogen gas (N2) by bacteria.
Humans have doubled the amount of available nitrogen (ammonium, nitrite, and nitrate) by fixing it through the Haber-Bosch process to produce fertilizers. When excess fertilizer runs off, it enters the water supply through streams, rivers, and lakes. Excess nitrogen in aquatic systems can lead to eutrophication, and ultimately dead zones, of which there are currently more than 400 throughout the world (an increase of more than 10-fold from the 1970s). Students may be familiar with local ponds or streams that are eutrophic, or very green, due to the excess algal growth.
Marsh plants, particularly the taller graminoid plants (common reed and cattail), are very good at removing nitrate from marshes, and wetlands can remove 85-99% of ammonia. Most wetlands are thus a “sink” for nitrogen, which means they absorb more nitrogen than they release. In the Hudson River, wetland plants remove nitrate-nitrogen from the water on the incoming tide, returning water that is lower in nitrate-nitrogen levels to the main stem of the river on the ebbing tide.
Extend
Hand out the short article from the Poughkeepsie Journal on the constructed wetlands at the Dutchess County SPCA. Ask students to discuss, in groups, why the SPCA decided to build wetlands when developing their new building. Answer: For the SPCA, the current facility couldn’t handle the additional wastewater that was projected with the new building, so the wetlands were created to treat the water naturally. They knew their water would contain a high nutrient load that would need to be removed in some way; wetlands are excellent at removing nitrate-nitrogen.
Evaluate– Students will hand in the completed lab. Pay particular attention to questions 7 & 8, which ask students to relate the nitrogen changes in the marshes to vegetation types and to apply what they have learned to a realistic scenario (the desire to enhance water quality without building a new waste treatment facility). An answer key is provided.
Standards
Benchmarks for Science Literacy
5A Diversity of LifeNYS Standards
MST 4- Physical setting, living environment and nature of scienceCredits
Mary Musolino